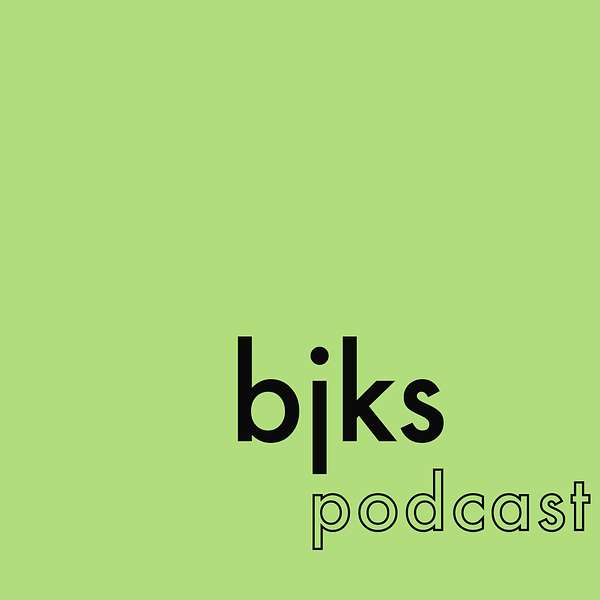
BJKS Podcast
A podcast about neuroscience, psychology, and anything vaguely related. Long-form interviews with people whose work I find interesting.
BJKS Podcast
15. Kate Jeffery: A brief history of spatial navigation, place cells & grid cells in 3D, and brain evolution
Kate Jeffery is a professor of behavioural neuroscience at University College London, where she works on spatial navigation. In this conversation, we talk about the history of spatial navigation, Kate's work on grid cells and place cells in 3D, and her recent work on entropy and brain evolution.
Timestamps
0:00:05: Kate's journey from medicine to neuroscience
0:10:57: A brief history of spatial navigation
0:30:43: PhD applications now and in 1990
0:34:38: Kate recorded grid cells 10 years before their discovery, without realising it
0:52:00: Prizes in science
1:05:20: A brief interlude as Kate gives her cat a treat
1:05:48: Lessons from working with Richard Morris and John O'Keefe
1:09:28: Spatial navigation in 3D
1:34:54: How many dimensions can the hippocampal formation track?
1:40:50: Kate's collaboration with Carlo Rovelli
Podcast links
- Website: https://bjks.buzzsprout.com/
- Twitter: https://twitter.com/BjksPodcast
Kate's links
- Website: https://jefferylab.com/
- Google Scholar: https://scholar.google.de/citations?user=l1VlIFAAAAAJ
- Twitter: https://twitter.com/drkjjeffery
Ben's links
- Website: www.bjks.blog/
- Google Scholar: https://scholar.google.co.uk/citations?user=-nWNfvcAAAAJ
References
Aronov et al 2017. Mapping of a non-spatial dimension by the hippocampal–entorhinal circuit. Nature
Bliss & Lømo 1973. Long‐lasting potentiation of synaptic transmission in the dentate area of the anaesthetized rabbit following stimulation of the perforant path. J Physiol
Burgess 2014. The 2014 Nobel Prize in Physiology or Medicine: a spatial model for cognitive neuroscience. Neuron
Casali et al 2019. Altered neural odometry in the vertical dimension. PNAS
Fyhn et al 2004. Spatial representation in the entorhinal cortex. Science
Grieves et al 2020. The place-cell representation of volumetric space in rats. Nat Commun
Grieves et al 2020. Grid cell firing fields in a volumetric space. bioRxiv
Hafting et al 2005. Microstructure of a spatial map in the entorhinal cortex. Nature
Jeffery et al 1997. Directional control of hippocampal place fields. Exp Brain Res
Jeffery & Morris 1993. Cumulative long-term potentiation in the rat dentate gyrus correlates with, but does not modify, performance in the water maze. Hippocampus
Jeffery & O’Keefe 1999. Learned interaction of visual and idiothetic cues in the control of place field orientation. Exp Brain Res
Jeffery et al 2019. On the statistical mechanics of life: Schrödinger revisited. Entropy
Jeffery & Rovelli 2020. Transitions in brain evolution: space, time and entropy. Trends Neurosci
Morris et al 1982. Place navigation impaired in rats with hippocampal lesions. Nature
O'Keefe & Dostrovsky 1971. The hippocampus as a spatial map: Preliminary evidence from unit activity in the freely-moving rat. Brain Res
Ranck 1984. Head direction cells in the deep layer of dorsal presubiculum in freely moving rats. In Society of Neurosci Abstract
Rovelli 2016. Seven brief lessons on physics
Shannon 1948. The mathematical theory of communication
Stensola et al 2012. The entorhinal grid map is discretized. Nature
Yartsev et al 2011. Grid cells without theta oscillations in the entorhinal cortex of bats. Nature
So in my extensive research by Googling Kate Jeffrey CV, I saw that you actually started off as a medical doctor, or at least you did medical training before you did your PhD. And I was just curious, how did you, uh, how did Kate Jeffrey in the late eighties? Um, in New Zealand, as a medical student in the late eighties.
Kate Jeffery:Early eighties, I'm sorry to say.
Benjamin James Kuper-Smith:80 to 85 here. Um, but how did you end up as a medical student, New Zealand then a few years later as a PhD student in Edinburgh working on, I don't know whether it was memory or spatial navigation, but
Kate Jeffery:Yeah, I was, I was working on, um, synaptic plasticity. Yeah. So it was a slightly circuitous route because I started, um, I started my scientific career doing medicine because I didn't think I could be a scientist. So I grew up in Dunedin, which is this little city in that far side of the world. And, um, my father was a surgeon and, you know, all of their friends were medical and none of us knew any scientists and it wasn't, it wasn't a career that really seemed viable in that environment. Um, so when I was trying to decide what to do with my life, I thought, well, I'll do medicine because it's scientific and I know it's employable. And, you know, I was very steeped in the medical world and it was very familiar and interesting. And so I studied medicine, but I always kind of knew that I wasn't very practically minded. And I was head in the clouds, intellectual, type, absent minded professor type, even as a medical student. And, you know, I was reading lots of stuff that wasn't really related to medicine. And when we had to, in third year, we had to do a project for our behavioral science course. And most people did normal projects that a medical student would do looking at, you know, studying people's behavior or their attitudes to illness or this, that, and the other. I decided to research information theory, um, which was this concept that I'd stumbled upon. Um, and so I learned all about, um, the, sort of the theory of communication. So, um, Claude Shannon back in the 1940s laid out the sort of theory of how information, um, could be contained in certain sort of formats and transmitted. And that, that sort of basically leads onto the brain and neurons and how neurons transmit information. And I thought, this is, this is kind of interesting. And then I came across this book by Douglas Hofstadter, who is a cognitive scientist, who has thought a lot about the mind and how the mind is generated from neurons. And, you know, there's this kind of simple units, which in and of themselves don't have minds, but when you put them together can generate minds. And so he wrote this book called Godel Escher Bach, which is about two and a half inches thick. It's weighty tome. And I started reading this book and I, I sort of took it with me on holiday. I was going hitchhiking around the South Island of New Zealand with my flatmate. I brought this book with me to fill in the long hours sitting on the side of the road in the baking sunshine with our thumbs out, waiting for a car to come along, or, you know, much of it, I remember sitting in a tent on this very sort of deserted beach on the West coast of the South Island, which is just this incredible location to be thinking about the mysteries of the mind. And I read the entire book from start to finish. And I just thought, this is the coolest thing I've ever come across. This idea that the mind is just a product of these, um, physical processes. And how does that happen? How can you make a mind? You know? And so, so it sort of started from there. I then went back to the hospital and finished my training and so on, but it was kind of preying on me that really, I want it to be studying this other stuff. And I thought, how do I do that? And so I decided to, um, a master's degree and see where the research is, the type of thing that actually I, I could do. So by now I had a degree in, I knew that I could pay the bills if I ever needed to, if I were doing medicine, but I thought I'd try my hand at, at scientific research. And so I went to the, the only neuroscientists I knew of which were in my hometown of Dunedin, where I had done my first part of my medical training. And I discovered that in the psychology department was this very young and energetic new professor called Graham Goddard, who was studying this structure of the brain called the hippocampus, which I had dimly come across, but didn't really know anything about. And he was interested in the way that the connections and hippocampal neurons can change in strength. And he said, yeah, why don't you join my lab for a year? And you can do a project and see how you liked research. And I was super excited because I thought this is my chance to really study the brain. And I thought, okay, well, first of all, I'm going to finish the plan I had already formed, which is go to England for a year and just, you know, do so young, new Zealanders will often, um, at the start of their adult lives, they'll go and spend some time overseas, it's called getting your OE your overseas experience. And then they'll go back to New Zealand. I thought, okay, I want to do my OE so I'll go to London, I'll spend this year in London and then I'll come back and I'll do my masters with Graham Goddard. And just before I went to London, I went hiking with some friends in the mountains of the South Island, which is very, very rugged inhospitable, um, kind of countryside, very steep, jagged mountains, very, very high, quite dangerous. And while we were in these mountains, like days from anywhere, um, we were caught up in this big storm and we spent a night huddled in our tents in fact maybe more than one night huddled in our tents with the rain lashing down and the wind, and, you know, the rumblings of rocks and mountains above us. And, you know, I thought we were going to die. And then the rain cleared and we kind of staggered out and went to Queenstown, which was the nearest towns where we were, um, you know, staggered into nearest cafe bought a coffee and newspaper sat down, thought who, you know, we made it open the newspaper. And there were on front page, was this story of a neuroscientist who had been caught in the storm while out hiking with his family and had been swept away in a river and drowned. And it was my would be supervisor Graham Goddard. And I was absolutely devastated. Um, there's a terrible, terrible tragedy. Um, and he left behind a wife and young child and also, you know, shattered dreams of all of the people working with him. And so, um, so, so I went to London and then I did my OE, while I was there, I got a, um, a letter. So in those days there was no email. So it was a typed letter from Cliff Abraham who was a colleague of Graham Goddard's. And he said, look, I remember when you came to visit. And, um, I remember that you wanted to come and do a master's, so that offer is still open. You can come and work in my lab, I'm working on this phenomenon also in the hippocampus called LTP, which we think is how memories are made. And I thought, Oh, that sounds great. So maybe I can, you know, maybe my plan hasn't turned to dust at all. And he also said, while you're in London, you should go and visit John O'Keefe, um, who was working on hippocampus. He's kind of an interesting guy. And so I went to visit John O'Keefe and at that stage I was, um, I was experimenting with, um, the eighties fashions, which I don't know if you know, the eighties fashions, but it was spiky dyed hair, uh, doc Martens. So, so I went to visit John O'Keefe with orange spiky, died here and, um, Doc Martins that laced all the way up my legs I don't know what he thought of me. Um, and sort of said, yeah, I kind of interested in the brain. I sort of want to, I want to figure out how it works. Um, and he was very sort of kindly told me all about these cells, he'd discovered called place cells. And I thought that sounds kind of interesting, and I sort of filed that away. So then I went back to New Zealand and I did do my masters with cliff Abraham. And I learned about LTP, which is, um, this way in which neurons, if they're kind of active together, then they will strengthen the connection between them. So this was an idea that was developed by Hebb, who, um, was working in McGill and, um, some of his students, um, back in those days, in McGill included John O'Keefe and also Graham Goddard and, you know, so there's all tangled web of interconnections. So I, um, so I did my masters on LTP and I sort of looking at, um, how it correlates with the, with the duration of memories. And at the end of that, I thought, this is, this is neat. I actually really love research. I want to keep doing this. Um, so what do I do next? And I was going to try and do a PhD also with Cliff. So I was going to carry on my, my master's work. But, um, the New Zealand medical research council, which was really the only route for funding ran out of money that year, they said, sorry, we can't fund anything. So this is, you know, this is my instinct that New Zealand is not a great place to do science was kind of born out by that experience. Um, so they, they said we haven't got the funding. So, um, so I thought, what am I going to do? And I came across an advertisement by, um, this scientists in Edinburgh called Richard Morris, who I knew of, because he was very famous in the field of LTP. And he had been trying to link it to learning. So I'd read all his papers and I thought, you know, this famous guy. So I wrote to him and said, look, I know you're advertising for a post-doc and I don't actually have my PhD yet, but I do have a medical degree and I really want to come and work with you. And amazingly, he took me on site unseen. So I traveled to Edinburgh and I did my PhD with him and learned all about LTP. And while I was there, I had the opportunity to visit Johnny O'Keefe's lab again, this time now knowing something about the area and I discovered place cells. And I thought, you know, LTP is interesting, but place cells are amazing. I want to study place cells. And that was the beginning of the rest of my career. Basically.
Benjamin James Kuper-Smith:That was a much more interesting answer than I expected. It was great. I thought it was going to be something like, well, you know, I did medicine, I didn't really want to do it. So I thought, well, I'll just
Kate Jeffery:So you did say you could edit this down, right?. And you did say I could wander off track and it doesn't have
Benjamin James Kuper-Smith:No, I'll keep that, that was great. I also didn't know that you'd already met John O'Keefe before even doing your master. I mean, that was really fascinating. And I like one question I, uh, also wanted to ask, which you kind of already answered is, um, whether Richard Morris was already as famous as he was, because, I mean, this is a general point, I'll, you know, I started learning about this stuff. 2014, I took Neil Burgess's course, literally three weeks in John O'Keefe won the Nobel prize. So like, I know that this is big stuff. Like this is, this is how I got to know it as something like this is really famous, really cool research. But I was one question I wanted to ask, like, whether, for example, Richard Morris, who was always spoken very highly in Neil Burgess's course was already famous then, but I, I think you already answered that question, right?
Kate Jeffery:Yes, he was. So by that stage, so this was the early so 1990 was the year that I arrived in Edinburgh. And by that stage, his water maze task was becoming quite widely accepted as the way to measure hippocampal function because the water maze task requires navigation. So I don't know if your listeners will know about the water maze task, but basically the idea is that you put a rat in a swimming pool about two meters across and it, um, it swims around until it stumbles on a, on a platform that it can climb onto. And can't see the platform because the surface is just below the surface of the water, but it can climb onto it and rest and shake itself down and so on and so on. And the only way that the rat knows where the platform is, is by looking at the landmarks that it can see in the room and the outside room. Because there's nothing in the pool that marks the location of the platform. So it's really a fairly pure test of the ability of animals to kind of integrate, um, a variety of spatial cues that are in different locations and use that to pinpoint a location that in itself isn't marked and it turns out to be extremely sensitive to damage to the hippocampus. So the landmark study that Richard Morris did with John O'Keefe was to lesion the hippocampus and show that, um, no, sorry, actually that predated, um, has worked with John O'Keefe. So he showed that if you lesion the hippocampus and the animals are very impaired at finding water maze platform, and then he collaborated with John O'Keefe and they looked at what happens if you block LTP. So this memory mechanism that I had been studying, um, with Cliff Abraham, um, if you, if you put into the brain, a drug that blocks that process and stops these connections between neurons forming, if you put that drug into the hippocampus, then you stop the animals, being able to find that hidden platform. So this was all kind of coming together to suggest that the hippocampus has got something to do with, with, um, processing spatial information. So spatial mapping of some sort. So, I mean, he became a lot more famous after that because he then went on to a lot of very elegant work, linking the spatial processing with episodic memory. So memory for life events. And, um, he did some very, I mean, he's known for his very clever experimental designs and, and also for his development of quite clever methods for finding out what animals know. Um, so he's really very skilled, um, behavioral neuroscientist, so both on the behavior side and the neuroscience side. So he was tremendously influential.
Benjamin James Kuper-Smith:And on, in that line, I mean, again, when I first learned about this in hindsight, this was all very famous and well known and very cool, but were place cells, I mean, so in 1990, I guess when you started was quite a bit before grid cells were found and around the time that the head direction cells were found, right. I think it was late eighties or something, but it was this already like as much of a, like, was that as much hype behind the few or was it just like a few random people doing these things and people didn't quite realize how important it was going to be?
Kate Jeffery:Yeah, I think it was the, the beginning. I mean, it's seen a sort of an exponential rise, I guess. So, the first paper on place cells was published in 1971 and throughout the seventies, um, it was really only John O'Keefe who was, um, studying these things and, and the papers were, um, fairly widely spaced in time and not widely accepted. So a lot of people felt, so place cells are these neurons, single neurons in the hippocampus that become active when the rat goes to a particular place in the environment and O'Keefe had stumbled on them. Um, I think sort of by accident when he was looking for, um, the role of the hippocampus in memory. So we knew at that stage, so back in the 1970s, we knew that damage to the hippocampus produces really profound impairments in episodic memory. And we knew that from human studies. And so the neuroscience community was trying to find a correlate of that and animals so that we could study this you know, the contribution neurobiologically. So Jim Ranck in the US developed a method for recording single neurons in awake behaving animals, and this involved, flexible microwires
Benjamin James Kuper-Smith:That they could move around right. That was the, was that the novel part that they could actually move rather than,
Kate Jeffery:Yes, that's right. So, so up until that time, when people had wanted to record individual neurons, the animals had to be anesthetized and immobilized and the, and the electrodes were rigid sort of glass or tungsten or something like that. So quite stiff. And, um, those electrodes turn out not to work very well if the animal can move around, because they're so rigid that the tiny movements of the brain as the animal is moving, just dislodge the electrode relative to the neuron, you, you can't, um, you can't record the same neuron, whereas with flexible microwires, they kind of are flexible enough to get carried along with the brain as it's moving. So they're much more stable. So that was quite a revolutionary technology. And, um, O'Keefe learned about this technique from Ranck. Um, when he set up his new lab in London, he started using this technique to record these hippocampal neurons. And that was when he observed that the neurons would often become active when the animal went to a particular place in the environment. And he called these neurons place cells, but it took a long time for people to accept that characterization of them, because, because place is quite an abstract concept. And at that stage, people were still thinking of the, um, the brain is a pretty mechanistic device that associates inputs and outputs with not too much other stuff in the middle. So, um, this was an idea that had developed in psychology in the early 19, so the early 20th century. So in the early 19 hundreds, um, this idea that the brain is basically forming connections between what goes in, what comes out. So it's, it's an associative network. The way of studying that behavioraly was a discipline in psychology, known as behaviorism, um, and in its strongest form, behaviorism claims that, um, that there are no processes that are not visible in the behavior of the animals. So there's no kind of black box process and going on somewhere in the brain. Um, it's all fairly straightforward connections between what goes in and what comes out. And, um, behaviorism, the strong form of behaviorism would claim that all animal behavior and all knowledge and all thinking could be explained with pretty flat associative network. So the idea that a neuron could be responding to something really abstract like place, um, as opposed to some sensory stimulus, like being able to see that door over there or that window or something like that. Um, it was just a bit too far from the associative behaviorist notion. And so it wasn't well accepted. And so O'Keefe was challenged a lot, you know, how do you know that the animal can't just smell some, there's some spot on the surface that it's walking around on. And so he had to do a lot of work, um, lot of control conditions, you know, showing that the place cell would still fire in the same place. If you change the floor paper, or if you replace the box with a different box, that looks exactly the same, or, um, or if you moved the entire box, a couple of inches to one side, uh, everything, you know, the place cells firing would shift a couple of inches as well. So it was, it couldn't really be explained by simple sensory inputs. Um, but you know, slowly the, um, evidence accumulated more and more people started to, um, to pick it up and to start to characterize the type of information that the cells use. So throughout the 1980s and the 1990s, a lot of experiments were done and not just by O'Keefe, but by other people too. So that, that also made it more acceptable people who were replicating the findings and, and seeing the same phenomenon in multiple labs. So that became more convincing. Um, and, and finding things like, you know, you can show that a place. So if you, if you have a fairly simple symmetric environment, like a cylinder with a single cue card, you know, it's just a white piece of cardboard on the wall or something. If you take the animal out of that environment, move the card around to a different part of the wall and then put the animal back. Then the place cell will shift its firing to follow that that rotated cue. So that tells you that the cell is getting some type of information from that visual cue. And so lots of, lots of experiments came along, um, showing how the cells are processing sensory information, um, and showing that they are indeed extracting, uh, this more abstract representation built up from the sensory information coming in. And one of the big insights was that the cells can combine static sensory information from the environment together with information about the animals' movements. So for example, you can have a place cell in a cylinder and it fires in a particular place. Every time the rat goes there, it'll become active. And you know, that that cell is responsive to a cue card because, you know, if you take the animal out and rotate the cue card around and you put the animal back in, you know, the cell, whatever of rotation is firing. So definitely it's getting sent this visual input, but you can also turn out the lights. So now the animal can't see the cue card anymore, but the place, so we'll still fire in that same place and it will do so for quite a long time. And the reason is that the brain can substitute, like while it's for that period of time, when it's missing the visual inputs, it can use the self motion tracking system in the brain to update its calculation of where the animal is. So even though the animal, can't see the cue card, it's your moment. The sense of direction, for example, has still maintained that it's tracking of which way the animal is facing. And it can do that for quite a few minutes. And then when the lights come back on and the animal can see the cue card again, if the sense of direction had gotten a little bit out of alignment, it adjusts itself. Once the animal can see the cue card, it's it switches back. So, so you can kind of switch back and forth between the internal sense of direction and the external visual landmark, and that, you know, again, that, that, that tells us that what the cells are processing is to do with these spatial characteristics place and direction. Um, it's more than just raw sensory information.
Benjamin James Kuper-Smith:What paper is that with the turning the lights off,
Kate Jeffery:There are a few papers. Um,
Benjamin James Kuper-Smith:So the reason I'm asking is also that I always put like papers, we talk about, I put in the description of the, I put the references in the description of the episode. So, and I actually don't know that paper. Yeah,
Kate Jeffery:There are, there are, um, there are sort of several looking at what happens. Some are looking at the head direction system and some are looking at, um, at place cell firing. And then there's the experiments that I did when I was in John O'Keefe's lab. So I went to work with John O'Keefe as a post-doc after my PhD with, with Richard Morris. And so one of the things that I was looking at is this interplay between, um, what the animal can see and what its sense of direction is telling it. And so what I found was that, um, so you can do this experiment where you take the animal out, you rotate the cue card and put it back and the cells will rotate. But the other thing you can do is you can take the animal out, put it in a little box, very slowly, turn the box around by, let's say 180 degrees and put the animal back in the environment and if there's no other conflict, so if you've taken the cue card away, so there's nothing, there's nothing to, um, tell the animal that it's wrong and it's assessment of which way it's facing. The fact that you've turned the animal round 180 degrees, will rotate the place fields by 180 degrees as well. So it's like the animal was carrying the direction of everything around in its head. Um, and then when you put the animal back in the environment, the place cells use that to establish their firing fields. And then I did some work sort of looking at what happens if there isn't that conflict between the, what the animal can see and what its internal sense of direction tells it is where it thinks it's facing. And you can switch the trust in that system between the sense of direction and the visual cue card by teaching the animal that one or other of those things is not reliable. So for example, if you move the cue card so that the animal can see that it's moving, then the place cells learn, okay. We can't trust that cue card, it moves around. So it's not a very good indicator for our sense of, you know, for our directional orientation. So we'll use the sense of direction as the, um, the rest of the brain instead. So you can actually see this dynamic updating and learning, um, of the spatial system based on past experience. So it's quite a plastic system, and that's what we think all of that LTP stuff is for it's for adjusting a lot of these connections and, um, helping system adapt on the best experience.
Benjamin James Kuper-Smith:Yeah. And was that then, was it, was that then your PhD, the relationship between LTP and place cells or was it
Kate Jeffery:No, my PhD was, um, it was looking at the relationship of, so w I was looking at water maze, um, behavior because I was in Richard's lab for that. And I was looking at the, um, correlation and individual animals between how easy it is to induce LTP and how well they learn the watermaze task. And I found this quite good correlation between those two things. So there's a lot of individual variability and plasticity, and that was reflected in, um, speed of, and durability of spatial learning. But, you know, when I then went to, um, work in John's lab to look at place cells, I carried with me this sort of interest in plasticity and learning and how that, how the system learns and updates. And, you know, I wasn't alone, obviously lots of it's, it's a very big area. Plasticity is, you know, tremendously important for forming memory and it's a big field, but I was sort of linking it to play cells. And, um, it's still an interest of mine.
Benjamin James Kuper-Smith:Okay. Um, I have one very silly question. Uh, did Richard Morris refer to the Morris water maze as the Morris water maze? Um, I really want that to be the case.
Kate Jeffery:I don't think he did. Offhand, I think he referred to it as the water maze.
Benjamin James Kuper-Smith:Okay. The water maze,
Kate Jeffery:Everybody else calls it. The Morris water maze.
Benjamin James Kuper-Smith:Exactly. Yeah. That's, like, I've never heard anyone say anything other than the Morris water maze.
Kate Jeffery:I don't think he used that. I could be wrong, but I'm pretty sure I'm not.
Benjamin James Kuper-Smith:Yeah, it would just be great.
Kate Jeffery:I was very fortunate. I also encountered Tim Bliss in my very early career. So when I was doing my master's degree with Cliff Abraham in New Zealand, we had a visit from Tim Bliss and we were, so he knew Cliff very well. They were old friends because Cliff had worked sort of, um, in Europe, in the early part of his career. Um, and in fact, I hadn't really realized, but I was Cliff's first, um, postgraduate student. I had no idea how young he was to me. He was a sort of grandfatherly figure. Um, and so Tim, um, visited Otago while I was there. And, and, um, so we had quite a few scientific discussions and, um, I kind of felt like I got to know him quite well. And so when I went to Edinburgh, I'm pretty sure actually that he put in a good word for me with Richard. And that was one of the reasons that Richard took me on sight unseen as a PhD student, but Tim, um, examined my PhD thesis. So I was tremendously privileged to have. So he discovered LTP. I probably should say that because it's not necessarily something, you know, but he, he discovered, um, LTP. And, um, in fact, he was working in, um, McGill with Hebb. So he's another one of these connections know. Um, actually I can't remember if he worked with Hebb or slightly after, but anyway, he was very influenced by Hebb's ideas and his idea was to try and see if he could see this connectivity forming between neurons. And he didn't manage to do that for his PhD. So his PhD was sort of a failure, but when he went to visit Norway to visit his friends, um, Terje Lomo, Lomo was studying, um, neurons in hippocampus. And he, he said to Bliss that you've got to, you've got to look at this thing that I've found. I found that when I stimulate these pathways into the dentate gyrus, part of the hippocampus, when the preparation gets a bit tired at the end of the day, if I give this high frequency burst of pulses, then everything gets much stronger. Um, Bliss said, look, this is, this is amazing. This is synaptic plasticity. This is what I've been trying to find in my PhD. And I was looking in the visual cortex, but here you found it in hippocampus. So, um, he and Lomo, um, went to London and, um, and got this working and they published this paper, which has had possibly one of the, one of the most highly cited papers in all of neuroscience, uh, reporting this phenomenon of LTP. Um, and LTP is it's now so well established that it's just that we just, we just talked about LTP. Like every, every neuroscience student knows what LTP is more or less without explanation. And so to have Tim Bliss come to New Zealand while I was, there was this incredible privilege and to have him examining my PhD was also this incredible privilege. And, um, so when I started applying for jobs, I was, I wrote to Tim and said, look, would you be one of my referees along with Richard Morris and John O'Keefe? So I had these three luminaries. Yeah. I, and I, I didn't fully, I think back then, appreciate just how incredibly lucky I had been to just fall in to this, this network of people who have just been pivotal, um, in our understanding of this system. And, you know, looking back this, this little kid from New Zealand with big aspirations to do science, you know, I just can't believe how, how it all turned out. And sometimes it's, it's amazing. So, um, so yeah, so, so that was, that was how I got started.
Benjamin James Kuper-Smith:Crazy the coincidence is here. I mean, as just from meeting the people randomly and yeah, that's pretty crazy. Um,
Kate Jeffery:Yeah, I think it does say something about the importance of networking and the importance of introducing young scientists to senior scientists in the field. And you know, that we have sort of mechanisms for doing that conferences and so on. And I think we need to remember as a discipline, how important those opportunities are in shaping people's careers and inspiring them, all of that sort of stuff.
Benjamin James Kuper-Smith:One question, I had something you said earlier that, I mean, that Richard Morris offered you the PhD position without, I mean, did he literally just get that one letter from you or was, was there any more to it or was it just your, that he said, okay, if you want to come
Kate Jeffery:Something like that. I mean, I mean, I think, you know, he, he must have, um, I mean he knew cliff Abraham, who's a well-respected figure. And, and, um, like I say, I think possibly Tim bliss, or he possibly also asked him to said something or something like that. But, you know, in those days, I mean, I was in New Zealand and Richard was in Edinburgh. Wasn't going to fly, fly over for an interview. Um, we didn't have zoom. We didn't, you know, we had telephones, I think we possibly had a conversation. Yeah, it did. Yeah. And, and, you know, um, so it was, it was definitely not the hoops that we make post-docs and PhD students dumped through these days to get positions,
Benjamin James Kuper-Smith:Ask, like, whether that's do you think that's a good system? I mean, like, because now it's a thing where you have to, you know, hand in all these things as CV, you meet the people, you have interviews if several letters of recommendation, et cetera, et cetera. Um, w I mean, what do you think are the advantages disadvantages of either system?
Kate Jeffery:Well, I mean, the system that we have now, I mean, to be honest, I think there's possibly still quite a lot of word of mouth, um, happens and networking and, and, you know, that type of thing, which is great in some ways, because it means, you know, if you've, if you've come across a really bright student, um, and you know, you know, a colleague who was looking for someone, um, and you can put those two people together, you know, in some ways that's great. But on the other hand, um, it does mean that you have this system where you're drawing a line between the privileged people who are, who have contact with those networks and the less privileged people who haven't. So, um, that's fine. A few, uh, if you stumble on a network and I, you know, I was in some ways quite privileged because although I was in New Zealand, um, I was in medicine. So, you know, that gives you some credibility. And, um, you know, I was in a sort of local network if you've got somebody who's coming from, you know, somewhere in South America, and hasn't really had the opportunity because of geographical distance, for example, to meet all of these people and to get plugged into that network, um, those types of people get left out. And the thing about the system where you advertise widely and you have shortlists and, you know, all of that kind of stuff, although it's a bit more tedious administratively, I feel that's a bit fairer. So I think, I think we do need to kind of modernize, modernize a bit, but there's always a place for networking because, you know, one-to-one interactions can be very inspiring. And
Benjamin James Kuper-Smith:I mean, also obviously an interview is not a letter of recommendation or someone saying, uh, Hey, this person is really like, this person is on research with me and is really good. Is worth a lot more than someone answering 10 questions. Well, in an interview, right. They're just completely separate diff situations doing research and doing an interview. So in some sense, it also, yeah, it does make a lot more sense.
Kate Jeffery:Yeah. It also allows for things like, you know, this person, um, didn't manage to get any publications from their PhD, let's say, but the supervisor is that, you know, maybe bumped into someone at a conference or something and says, I've got this really amazing student. Unfortunately they have not published anything. So their CV doesn't look great, but that's, you know, this person that's super smart and extremely talented and blah, blah, blah. So, you know, I don't know. I mean, the, the, the word of mouth thing also has some benefits. I don't really know what the answer is to the correct balance between those things. I don't know if there is one it's always difficult, isn't it?
Benjamin James Kuper-Smith:Yeah. Yeah, exactly. I wasn't expecting you to solve the problem. If you don't mind. I'd like to ask a bit more, a little bit about the, like history of the whole, of the field almost. And, uh, one way in which we can maybe do this is that, um, a while ago you tweeted a tweet about something that happened in the nineties. Um, so, so the date that you tweeted about is the 12th of April, 1994. I don't know whether two things that seem to happen on that day. The first is I celebrated my third birthday, not actually from UCL. Sorry, sorry. But, um, so that's one thing that happens. Another thing that happened is so, um, I think I just described the tweet and everything. You can explain what, what the significance behind it is. So the tweet is a photo of a seems to be like a piece of like a full paper onto, which is with like yellow adhesive tape, you kind of not clued, but would you say glued to it? Yeah. Sellotape to it, a small white square of paper, basically. And on that white square of paper are lots of black dots or black and white checkered dots. And those dots seem to, they kind of cluster. So they had, they're not randomly on this, on the square, but they seem to kind of cluster in three different points towards the top left top. Right. And kind of towards the middle of the center of the bottom and on the other, as you can see, it's his place. So, um, uh, the top of it or to the left of it. And then your, your tweet is, uh, sometimes when I'm feeling wistful, I like to take this out, look at it and cry medial and trying to cortex 12th of April in 1994. So would you like to elaborate on why this makes you cry?
Kate Jeffery:Yes. So, um, so I went to, um, Jonah Keith's lab as a post-doc in 1993. Um, so it's finishing up my pH D with Richard and then I moved and I was interested in place cells. So I discovered, you know, play, I had visited John John's lab and thought, I want, I want to study these, um, these cells. And John had this very accommodating attitude, which was when people come into my lab, they can study whatever they like, but like, basically, um, so which was nice in some ways, but it just kind of leaves you wide open to, um, how do I figure out what to study? And so I thought, well, what's the question about play sales? The question is, how does a place I'll know where to fire? So in a play. So, you know, when, when the rat goes to a place in the environment and the cell becomes really active and fires, all these action potentials, how does it know to do that? It's just a cell in the brain. That's just got others, other action potentials coming in. And so, um, so what we really need to do is to record from the inputs to that region and find out what are they doing. And one of the big inputs to the place of origin and the hippocampus is this region called into rhino cortex. And it's a part of the cortex that is connected to quite a few other parts of the brain. So the cortex is the sort of outer part of the brain. So the internal cortex is getting inputs from the visual part of the brain and the auditory part of the brain, all sorts of regions and kind of funneling this information into the place else. So I thought I'm going to try and record and from, from this region, and it's really difficult to get to because in the rat, it's right down the sides of the brain, it's very inaccessible, but I spent quite a lot of time trying to figure out how to get electrodes in there in a way that I could record
Benjamin James Kuper-Smith:What exactly is the difficulty. So
Kate Jeffery:The difficulty is because, um, because it's one of the side. So if you're recording from the hippocampus, which is at the top of the brain, you can make a hole in the skull. So you need to taste the rat, uh, make a little hole in the skull and put your electrodes and then seal everything up and let, let it all heal up. If it's around the side of the brain, the skull is much thicker and it's really hard to reach it's behind the ears and all sorts of things. And you can't just because it's inside, you can't just stick an electrode in. So I ended up having to go in from the top and angle the electrodes in the sort of quite difficult way. It took a bit of fiddling to get it to work, and it didn't always work. So I was just sort of starting to, um, to get this to work. And, and then when I did get my electrodes into what seemed to be the[inaudible] cortex, I just saw lots of sort of stuff happening. And it was quite hard to figure out what was going on. Lots of cells that just active all over the place. I did see quite a lot of cells that had a lot of rhythmic activity. And so I thought, well, that's kind of interesting. Um, and every now and then I would see a cell that seemed to have some sort of spatial activities. So its action potentials, weren't distributed all over the environment. They would be sort of in clusters, like, like with place cells and occasionally there would be multiple clusters. And so what that piece of paper shows you that was in the tweet is one of these cells that I found. Um, and it was a recording that I'd made that showed that there seem to be these three locations in the environment where the cell would become more active. And so I kind of looked at that and thought, well, this is sort of like a place, so, but messier, you know, um, and I saw a few of these things, but not very many. And meanwhile, I saw lots of these rhythmic cells and, um, and th they had some interesting properties. Um, so I thought, well, I'll, I'll just kind of write up a little paper on those and then I'll kind of move on. Cause I don't really have a handle on what's going on here. So while, while I was in that phase of my experiments, these two people came to visit the lab called[inaudible] Moser. And they had been doing their PhD in Trondheim, in Norway, um, with pear Anderson, who's one of these kinds of luminary figures in, um, LTP and plasticity and, and so on. And they had been working on plasticity. So I, so I knew them. In fact, when I had been doing my PhD in Richard's lab, they'd come to Richard slaps, I'd met them and I kind of knew them and knew that they were, they were, um, very super smart and super energetic. So we met again when they came to Richard's lab and they spent six months there and my job and that of my husband, Jim, was to teach them how to record play cells. So we taught them how to record play cells. And, um, Jim was an engineer of fact, and he, um, he had developed together with a technician in the lab, cliff Parker. He, um, he had built this new recording sort of system. And so the Moses said, okay, so we think place, I was a really cool, we want to study these. And we like the system. It's really nice recording system. It's using this new digital signal processing technology. So it's much smaller than previous recording systems and you could record lots and lots of channels and then, and on, um, can we buy one of your systems? So, so Jim actually formed a company and that company sold them a system and they went back to Toronto and started recording, no doing the
Speaker 3:Thing that there was the obvious thing to do, which was to record from the region of the brain. That's sending all these protections to the place cells and they were collaborating with Menno Witter. Who's a very talented anatomist. And he said, yes, and rhino cortex as where he wants to go. And this is the exact region of intrarenal cortex, where you should go, if you want to find out, who's talking to the place cells, this little region here. So they put their electrodes, um, in that little region there. And this was, um, experiments done by Mariana, from taco half-ton, who were two PhD students and then app. So I, I, um, met Mariana Fern and taco half-ton at the society for neuroscience meeting in 1994. And they had a poster showing that when they recorded from this little region of inter-annual cortex, they saw these multiple regions of activity and they then published this really nice paper in science and, and, um, Oh, hang on. No, it couldn't be 1994. It was later 2004. Sorry. I've got my decades. Well, it was 2004. So that's been 10 years sort of getting this to work and, um, recording these neurons. I mean, I did lots of other stuff in that 10 years as well. It was an amazing golden decade for that, that, um, but anyway, they had this poster in 2004 and they had these, uh, enter vinyl cells, but these multiple regions of our activities. So it was clearly something special going on and they published this nice paper in science showing this. And then in 2005, the bombshell paper came out and this was a recording from the same brain region, but in a much larger environment. So what had happened was they and other people, um, including bill Scaggs, who was a theoretical neuroscientist, had looked at the sort of pattern of, you know, blobs, um, and said, look, it looks like these regions where these ends rhino cells are firing are not randomly distributed. So although there are multiple regions in the environment, they're not like random multiple regions as if you're recording several places at once. For one thing, the regions are all really circular, unlike with place cells where the regions are often quite elongated or quite irregular, these are very regular. And also the space in between them is very even, and it looks like it's non-random. So to get a handle on this, the, the, uh, the Moses, so Mariana and talk on, uh, recorded in a very large environment, two meters by two meters. So a huge environment for a rat, and sure enough, they found this very regular pattern where the locations where the cells fire. So what's called the firing fields evenly spaced and arranged in these really neat rows that look like, um, like a grid pattern. So they call these cells cred cells. And I remember when I saw the paper of grid cells, it was one of those jaw-dropping flashbulb memories for me. I remember where I was, you know, um, and so on and so on. And, you know, it was just an extraordinary finding. Um, and the reason that was extraordinary is that the regularity of the pattern cannot come from the environment. There's nothing in the environment that has this regularity it's purely internally generated. And yet it also comes about because of the environment. So it's an interaction between something that's going on in the rat's head and something that's happening out there in the world. And it's really, you know, an incredible discovery. So, um, I realized in retrospect that my cell that I recorded in 1994 was one of these cells. So I go, I dug out my, you know, little bit of paper, um, and I realize that it's a very characteristic, you know, try and get a pattern, three blobs, evenly spaced. And I realized that, sure, that was a grid cell. Um, and I'm not the only scientist to have done that. Quite a few scientists who were kind of also asking the same questions. So Jim[inaudible] and other people, um, if you talk to them, they'll say, yeah, we, we now realize we were seeing things like this too, but nobody put it together until the Moser paper. So that was just incredible. And, and, you know, as you've mentioned, um, the Moses and her Keef together got the Nobel prize a few years later for the joint discovery of grid cells and place cells. You know, also I think a lot of us think head direction cells should be in there as well. So head direction cells, isn't this kind of th I, I think of it as the third leg of the stool of spatial cells, but, um, yeah, it was, it was certainly a very exciting decade to have been active
Benjamin James Kuper-Smith:Was, um, so one thing I think after they won the number press, I think near bird chest wrote a review of the history of spatial navigation or something like that. And I think in that he mentioned that main Avita also suggested to the Moses to use a bigger box, I think, or what do you call it? Not box, but, um, yeah, I read it every day.
Speaker 3:I think several people people said, so we had a journal club, um, about the 2004 paper. Um, and I actually, um, remember vividly that Tom Hartley, um, who had been working with Neil. And so he did his post-doctoral work with Neil and Tom said, I wonder if those, you know, blobs are, they, they look regular to me as well. And in fact, some of the, um, people in my labs of which Caswell Barry was one and Robin Haman was another, um, and my Michael Anderson. So, so three people in the lab at the time started trying to record into mine on neurons to see even before the grid cell paper was published. Um, people were looking for something along those lines. Um, but Marianne and talk quite there first. And, and the, the other thing I should mention is that the recording system that they made that discovery on was the recording system that Jim, my husband had had sold to them when they were in the lab. And so that recording system now resides in the Nobel museum in Stockholm. So that was a really nice kind of, you know, little connection for me. So although I, you know,
Kate Jeffery:Didn't get a Nobel prize for discovering grid cells. I came so close, you know, but we still, as a team, we were kind of in the novella museum, but, you know, I, I don't, I don't, um, I, I pretend that I cry over that picture, but I actually, I don't begrudge them that discovery because the amount of work that went into actually making the discovery and not only, not only discovering them, but they also did an incredible amount of work to characterize these neurons and to figure out all the connections. And it's just an astonishingly beautiful piece of, um, research. It's just science at its absolute best. And I, you know, I think it's an amazing, you know, one of the landmark discoveries of the century, I thanks the play cells and the good cells for helping us understand how the mind comes about really.
Benjamin James Kuper-Smith:Yeah. I mean, I'm also curious about the whole, I mean, like in, in hindsight now, will you make of the data you recorded then, and kind of like potential reasons kind of, almost more to meta level, like of, you know, why you didn't find the pattern? Like, was it that you didn't have you said earlier, you didn't have many spacial neurons, so whether just not enough spatial neurons for you to see that like triangle thing, um, was that part of the problem, or did you have different, I dunno, other arenas always kind of what they're always standard sized apart from those that were then bigger or
Kate Jeffery:Yes. So, so we tend to, so I mean, something that I've kind of noticed a lot in sciences that, um, that the discoveries get made when you just kind of do something, um, different from how it's normally done. So normally we would record in a, you know, an, a box that was small enough that that was big enough so that you could see these place fields. So play a typical place field in a rat might be like a few tens of centimeters across, or maybe even just a few centimeters across. It depends. Sometimes some of them are big and some of them are small. Um, so, so this is sort of trade off. If you make the environment too large, then the, the rat gets tired and bored and, um, full of rice before it's kind of covered the environment, densely enough for you to be able to see the, the pattern emerge clearly. So this is this trade off between getting good coverage, good even coverage, and getting, getting big enough coverage. And so the trade off is typically you have an environment's about a meter, and it just turns out that, um, the smallest grid, the smallest spacing between greenfields is about 30 centimeters. So in a meter sized environment, you just don't see many of these. And we just never had seen enough of them, um, for, for the penny to drop basically, but it emerged in a two meter environment. Then it became very obvious. And, you know, w we know she wants to record cord cells. Usually you'd use a meter and a half or two meter size box. Um, but it is hard work. It's hard to get coverage in a,
Benjamin James Kuper-Smith:I mean, they always throw around like food for the rats. So they just have some, some reason for walking around. Right.
Kate Jeffery:Yeah, that's right. Because you're trying to get the rat to do something that's unnatural because a normal rat would never spend all of his time in the open, like that certain your rats would normally follow quite stereotyped trajectories, you know, rat runs through the environment and they would venture out into the open to grab something, but then that scuttled back to somewhere safe. And so, you know, what you asking him to do to forage in this homogeneous way across a big open spaces, kind of unnatural. And, um, it's also, you know, they, they end up eating quite a lot, a few, even a few try. And, um, so, so typically we'll use rice or something like that, but just got a few calories and it tastes good, but, you know, we try not to fill them up too quickly, but even so by the end of 20 minutes or 30 minutes, they're getting a bit full and they'll want to sit in a corner and groom and clean up and Burton, perhaps have a nap, you know? So that's that, and the problem with behaving animals, you kind of have to work with the animal to some extent and, um, bribe it to do things.
Benjamin James Kuper-Smith:Yeah. But is this, I'm trying to, um, I mean, you said earlier, like one, one way that people make big discoveries is by doing things differently than they were done before. And trying to just kind of find some more like some general principles, like from that experience of you seeing many people doing this thing or trying to do the similar thing, and then it working only for one group, is it just questioning the assumptions as in like, this is the arena? Um, or, yeah, I'm just curious, like, whether there's anything you particularly like learned from that experience that you use now in your research, or,
Kate Jeffery:Um, yes. I think one thing is sort of question the assumptions or, you know, just, just deciding to go outside of the box a little bit, you know, there's, there's, it's, it's kind of a, trade-off, there's a, there's a risk to doing something differently that it's, that, that it won't work. Um, and that the reason that people do things the way they do this for a reason, because, you know, other people have tried this other things as well, and that didn't work. And so, so you're, you know, but on the other hand, if you do do something different, then that opens up opportunities to see something that people haven't seen before. So for example, the switch from tungsten and glass micro electrodes that I mentioned earlier to wire electrodes, um, enabled new types of recordings to be made, but you know, that, that it, wasn't just a matter of, Oh, let's try this different thing. Oh, it works. I mean, it took months and years of getting it to work, um, because it's, it requires different, um, a different approach. And, you know, you've got different technical problems that you need to solve. Like, how are you going to get the connection to the recording apparatus the animals moving around and how do you move the electrodes through the brain? And if it's not a tightest and immobilized and so on and so on. And that requires the development of like drive technology. So one of the big things that John O'Keeffe did with his, um, he had this very talented technician and an Ainsworth who developed, uh, uh, technology for moving electrodes through the brain. Um, so that opened up this opportunity. So it wasn't a simple thing and it required a lot of persistence and a lot of perseverance and, um, resources, and also the belief that that was going to pay off in the end. Um, and so, you know, it looks these new discoveries, they look like they're just happened, but really they're built on the back of a lot of groundwork. And the other thing is that we tend to attribute discoveries to individuals, but when you know the backstory, so, you know, we've attributed place else to O'Keefe and grid cells to, to the Moser lab and so on. Um, but when, you know, the backstory, you realize that really there's a huge number of people were involved. Um, and so it's like, I think of it sometimes it's like conquering Everest. You know, it wasn't one person that first conquered Everest, actually it was many, many people, including a team of Sherpas, um, who helped those climbers get there. And there's, you know, one or a couple in case of Everest, there was a couple who, who, who claimed it together, but actually it wasn't just those two people either. It was, you know, a whole huge community that made that possible. Science is exactly the same. And one of the problems I think we have with awarding prizes is that you single out some lucky people who got to be the one that got boosted above the, above the threshold. Um, but, um, what happens to the others? How do you recognize? And so I think it's important when you tell the stories to, to also look at the backstory. And often that's really interesting, and that's more interesting than the life story of one person, there's life stories of all of the people, um, that enabled those discoveries. And so I think the history of science, science, historians, and, um, popularizes of science, uh, incredibly important to the whole enterprise. And that's why I actually like to give up time to public communication of science, you know, how it all works. And, um, I like to do things like podcasts and lectures and tell people about the, the backstory, because it's, um, you know, it reveals the,
Benjamin James Kuper-Smith:It also makes it apart from showing the contributions of the, all the other people who apart from like the three or four, whatever, I think it also shows in some way, not, I mean, not only the contribution of others, but also often how special, the contribution of those people who did make it like that. They did, you know, as you said, like the Moses didn't just have this one paper where they found the grid cells to head also all this other stuff, characterizing it. And they, you know, they did do something differently when they
Kate Jeffery:Yeah. Yeah. But also, you know, they were able to attract exceptionally talented researchers. So Mariana Fernan, Toco half-ton, for example, the people who made the discovery, um, you know, they were attracted to the Moser lab because they knew that there was an excellent lab, but also it was an excellent lab because it had people like that in there. And I think we need to remember that as well, that, um, that it's, that these things kind of build on each other and we've got to not forget, you know, um, particularly I think we need to not forget those two individuals because, um, they didn't get the Nobel prize. Um, but technically speaking, they, they made the discovery. Um, but the, you know, so the prize really, if it was up to me, I would award the prize to a discovery. So the discovery of good cells and then, um, or the, you know, the discovery of whatever, um, relativity or something like that. Um, because also, you know, Einstein gets credited with relativity, but if you look at, um, how Einstein, um, came up with that again, you see that there's a whole bunch of other people involved as well, and he couldn't have done it without them. And so if we awarded the prize to the discovery, then, um, everybody involved in that discovery could feel part of that. Um, but of course, it's not up to me. To me, Nobel was Alfred Nobel who specified the price, but I would like to see, you know, discoveries get prizes,
Benjamin James Kuper-Smith:But isn't that kind of, I mean, so I agree, like, especially in the popular press and that kind of stuff, the focus always on the individual who made a contribution, but I think as soon as you know, a bit about this whole thing, and I think it's addressed quite a lot these days, that prizes are given to one person rather than even though lots of people contribute to it. But I feel like it is kind of like, I don't know my understanding of these kinds of prices, especially in enterprise is kind of that it is that like, it's, you're kind of awarding the discovery and symbolically some people stand in for that. I mean, sometimes it's yeah. So I feel, I dunno, I feel like that to me, at least that kind of isn't it, but I understand that often it's just, this person won the Nobel prize because of this experiment and a story.
Kate Jeffery:Yes. I, I, I sort of agree. I think that the Nobel prize, it sort of personalizes the discovery as well, and it, and it does acknowledge the extreme talent. I mean, you know, of course the people who were Nobel prizes, um, are exceptional scientists near-field, or occasionally they're just exceptionally lucky, but usually they're exception talented and lucky, you know, both together. Um, the problem is though that, you know, history then, um, you know, because history is, is a huge kind of diffuser, we lose all of the detail and we only remember the pinnacles. Um, and then all the other people eventually get forgotten. Um, and one of the things that we're starting to realize now is that, um, particular groups of people are more likely to get forgotten. So women, for example, and other minoritized groups who, um, turn out when you look at the history to have been incredibly important, but, um, because of human nature, didn't get the prize, you know, so, so you have to sort of think, how do we, how do we, how do we kind of, um, fairly attribute credit, um, and really the scientific enterprise is such a community thing. And also, I suppose, another, another issue is that by awarding prizes, to individuals, we convey the impression to the public, that individuals and individual discoveries are what science is all about. And so the public will often read a newspaper article that says, you know, scientists have discovered X when really what that means is a scientific group, published a paper about X, but lots of other papers didn't find X. And we're not sure whether X is a thing and it's going to require a lot more work to find out whether X is the thing, but yeah, yeah, the public, the public view of sciences that science has made by individual discoveries. And when we feed into that with our awarding prizes. So I don't know, I, I'm kind of, again, I'm a very split it's that same thing, but how do you,
Benjamin James Kuper-Smith:Oh, that seems to me that, from what you said earlier, it does seem like John O'Keeffe was someone who kind of, uh, went against the grain and that it does from at least from the, from what I, I mean, I pretty much the history mainly, no, what you've just told me, um, of trying to keeps work in particular. I mean, it seems to me there that he was, it wasn't lots of people working on the same thing and it was obvious that one person was going to get there eventually. Um, it, you see what I mean? Like, it sounds like he kind of maybe was one of those figures who advanced the field quite a bit.
Kate Jeffery:Yeah, I think so. I mean, the question is, you know, would play sales had been discovered anyway, and I think they probably would have been, so, so Jim Ronco had mentioned as, as, um, developing the, um, you know, the, the flexible microwave technology. He was pretty close to, to finding them because he was also applying the technique that he had developed, um, to the hippocampus. And if you read his papers from around that time, he was, um, very carefully trying to characterize what those neurons would respond to. Um, but he didn't, he didn't recognize that place was what they were responding to. And the question is, would he have done, had he had a bit more time? Um, maybe, maybe he would, maybe he wouldn't. So it's hard to know he did then discover head direction cells and, you know, he, so he, um, is credited with that work, even though he didn't, he didn't, he wasn't the first author on the first head. And so paper.
Benjamin James Kuper-Smith:Yeah, it's funny, isn't it? I remember one as tollway because it's pitching in German, um, like just literally[inaudible] um, but who are the other two? Always it wasn't it three authors or something?
Kate Jeffery:Um, uh, gosh, no, I think, um, John QB might've been one of the others involved. Um, so yeah, there were several authors, um, but you know, rock was the person who first reported the observation in 1984. And then Toby was the post-doc who, um, was sort of given the head hydration, sell projects, you know, find, find out what these cells do. And, um, so he was the first author on that paper in 1990, and that's a tremendously important discovery as well. Um, and Tabby then went on and did an amazing job of characterizing, um, many of the, you know, the sort of sensory correlates of hydration cells. And, um, he's been very influential for me because he was the first person who started to ask the question about the three-dimensional, um, properties of these neurons. So yeah. So getting back to, um, whether people who can be credited with the discovery, it's sort of hard to know, cause we haven't got the control and conditioner having that person make that, that discovery. But yeah, no, I think it does. It is, it is nice to recognize the tremendous, um, important contribution. And the other thing is, um, that, that, that just the hope that you might be one of those people and I say about science, which makes it unlike other competitive fields like athletics, you know, if you are not an Olympic athlete, you know, that you will never win Olympic gold medal, whereas there's always a hope and every scientist that you might want to know about price because you might get lucky. Um, so, so I think that that's quite a nice sort of thing as well.
Benjamin James Kuper-Smith:Yeah. I think as long as you're aware that it's a bit of a lottery, then it's fine. It's yeah. It's not deterministic. It's probably mystic and yeah. Then I think it can be a kind of nice game almost.
Kate Jeffery:Yeah. And it brings great joy to the field. So the, you know, the place of good sell Nobel prize really invigorated. I mean, we were just, everybody in the field was thrilled. Almost everybody I'm sure. One or two people, but, um, you know, basically it was just super nice to have that, um, acknowledged as an, as an important thing in bioscience.
Benjamin James Kuper-Smith:Yeah. I mean, that's also what I felt because you know, I came from psychology and you're a little bit about cognitive neuroscience from the kind of stuff they tell you in a basic psychology bachelor's in the UK. But then I, when I was at UCL and Neil were just in Castleberry module on, I mean, it's called neuro computation, but it was effectively half of it was on special education as an example of that. And then John O'Keeffe won the Nobel prize deck three, four weeks into that module. It was like, Oh, what we're doing is really important. I mean, I liked it before, but like, it's like actually like you get this kind of external validation, like this stuff is actually very important. What you do, what the teaching you right now. Yeah.
Kate Jeffery:Well, my, um, my cat has just walked in to say hello. He's hoping they'll give him a treat
Benjamin James Kuper-Smith:If you have one.
Kate Jeffery:Um, yeah. Do you mind, sorry about that. You can edit that out.
Benjamin James Kuper-Smith:I should put like some elevator music or something please send by Jeffrey.
Kate Jeffery:Yeah.
Benjamin James Kuper-Smith:Um, so I wanted to talk about the 3d stuff in a second, but before we do that, I just wanted to like, kind of finish off a little bit, the, um, your PhD postdoc experience with Richard Morris, Jonah, Keith. I was just curious. I mean, they both have, as you said, uh, achieved quite a lot. Um, is there a new work, so, you know, for, I guess the first several years of your research career in that abs, so I'm assuming they were quite influential in how you do research. Um, is there anything in particular you kind of learned from them or that you realize now like, Oh, I'm doing it the way they're doing it or,
Kate Jeffery:Yeah, probably probably. Oh, well certainly I guess something I learned from both of them, um, particularly Richard is his experimental design. So he, he is very good at, um, at really crystallizing a hypothesis and figuring out how, how to test it with all of the controls that you need to rule out the other possible explanations of things. And I sort of learned a lot just from designing experiments with him and seeing how he does it that I think has influenced me a lot. Um, although, you know, often the work that I do is a bit more exploratory. It's a bit more, you know, just what happens in this situation. Um, but you know, when I'm reviewing papers or if I'm, um, really wanting to kind of nail an observation, it's always in the back of my mind, you know, what are all the various compounding factors and how do I control for those? And so, and then, um, from John, I mean, I learned a lot from John and I guess one thing is, so he's, he's quite sort of ecologically minded. So thinking about what are these neurons doing for the animal, given the normal life that the animal lives and, you know, how do you get an animal to, to express its natural behaviors so that we can study these neurons doing what they do. Um, and I think I've taken that with me as well. I tend to think of about plus cells almost, almost in sort of psychological experiments, you know, what can the, what can animals see? What's it thinking about? Um, is it thinking about what you think it's thinking about I'd be doing something else, is it distracted? Is it, you know, what's its attention focused on all this kind of stuff. So, you know, thinking of these as part of a system, um, in an animal what's in a real world, and I, I guess that may be, what's sort of partly motivated my studies of complex space and sort of more naturalistic space, um, trying to, um, on the one hand, keep everything well, enough controls that we can answer meaningful questions, but also, um, looking at the world is, um, less stereotyped than, than the typical liberatory space.
Benjamin James Kuper-Smith:Yeah. I think it's really easy to get lost in your task. Right. And not always to forget like what you're doing this to study some actual behavior.
Kate Jeffery:Yeah, yeah, yeah. I think Richard Morris was also very much like that sort of, um, finding laboratory versions of real world problems that, that animals have to solve. I mean, not everybody is like that. Like there's a lot of research involves very artificial tasks, you know, like lever pressing tasks for rats and so on things that are kind of practically easy to implement in the lab, but it's sort of hard to think of, well, when would an animal actually do this in real life? You know? Um, so yeah, so,
Benjamin James Kuper-Smith:Okay. That's true. Um, okay. So as I said, I'd like to talk about your, you have two recent papers on, well, it turns out more than two papers, but there's two papers that I've read about especially navigation in three dimensions. Um, one thing that really surprised me is that, so I don't know. So when I took a few courses that related to these things, I always wondered like what would happen in 3d. And somehow I seem to have missed that there is already a literature on this. I read somehow I thought, I don't know, maybe I thought I looked this up and couldn't find anything, but just hadn't looked it up or something. But when I read, so you have these two, um, papers that I think almost published in nature communications last year and the other Canada's pre-print again, this will be in the description that the references, when I read those papers only realized just how much stuff and you in particular had done on three dimensional work, uh, rather than seeing like whether these, these phenomena of places and grid cells that we, the fast majority of studies observing 2d, whether they also play in 3d. Um, yeah, I just hadn't been aware that that already existed. Um, could you maybe briefly summarize kind of the, what you founded those papers and kind of maybe like what existed before and kind of what you added to that with these papers?
Kate Jeffery:Sure. So, I mean, the basic question is when you record an animal walking around on a flat environment, you get these regions where the play cells and grid cells fire, and when the animal faces in a particular direction, you know, hydration, solar virons on. So we've, we've kind of
Speaker 3:What, what I think of as a flat map. So the animal is just walking around a flat surface and we can see these sort of regions, which we think are what we call a cognitive map. And so the question is if the animal isn't walking around on the flat, what happens to the map? Um, because most environments aren't perfectly flat, very few animals live in a completely flat world, and there are sort of various possibilities. One is that the, that the map follows the surface of the environment. So if you're walking up and down Hills and valleys, and so on that, that the map just kind of follows the surface, um, or the other possibility is that the map is actually kind of three-dimensional in as if you could move through volumetric space. So imagine that you could fly or swim and you could, you could therefore go in any direction you wanted. So you could think of the map as filling this volume. And so if an animal was able to explore that space, you might imagine that the place where a place so would fire. So the place fields might be a volumetric structure, like a globule in space, if you like, and we're kind of cutting through this, globule when the animals walking around on the flat, but if the animal could move around in three-dimensional space, maybe we'd see this globular, um, kind of region. And similarly with the grid cells, we know that on the flat, the cells have this very regular, um, arrangement of firing fields. If the animal could move through volumetric space, would we see that this arrangement, this regular arrangement continued into the vertical dimension? So would there be a, a lattice of greenfields, each one, like a tiny little sphere and it all kind of stacks together. So, so I started asking that question a long time ago, by creating an apparatus that wouldn't let animals climb so they could get off the horizontal plane and they could climb in vertical space, but we hadn't yet figured out how to get rats to move through a volume. So it was just, let's get them to walk around and it sort of vertical plane and see what happens. And so we created this thing called the pegboard, which is like a climbing wall for rats. It's a flat surface with pegs sticking out of it and the rats can climb over these pegs. Um, so they can get off the ground. And the question is, you know, if a play cell has a place field on the ground, if the rat goes above the ground, will that place field still be there? Like, will it still have the same horizontal coordinates, even though the rat is now above the ground and similarly with the grid cells. So if, if there's a grid pattern on the floor, if the rat can be above the floor, will that pattern continue? And what we found is that the grid cells, so the plus cells seem to, they, they do have firing regions that seem to extend vertically as well as horizontally. And they're a little bit elongated vertically. So they're a bit longer in the vertical dimension than they are so that the tall of an hour wide as it were very slightly. Um, but nevertheless, they do have this kind of circumscribed shape. So it looks like the place cells are tracking how high the rat has gone as it's walking around on this pegboard, but the grid cells don't seem to track height. So they seem to just fire. So if there was a place on the floor where the grid cell would fire, it would fire at every height above that location provided the rat remained in that location. And some of the, if there was a place on the floor that the cell was silent because it was between two place fields, um, two grid fields, um, it would continue to be silent no matter how high the rat was. So the rat wasn't going in and out of grid fields as, as it got higher and higher and higher. So that, that made us think maybe the SMAPP is, um, well, a it's different for grid cells and place cells. So the place cells seem to be able to track height and the grid cells on. So that was highly surprising. And also the grid cells don't seem to be tracking distance moved in vertical space out. They're only tracking it in horizontal, but we also thought, well, the peg board has a slightly strange arrangement because although the animals and vertical space, it's, it's still oriented horizontally because it standing on the pegs. So its body is still horizontal, still horizontally aligned, even though the rat as a whole is, is up above the ground. Now, so maybe the grid selves are caring about the location of the plane of the body and because it's still oriented horizontally, they are acting as if the rats on a horizontal surface and they're not caring about how high it goes. So what would happen if the rat was actually oriented parallel to the wall instead of standing on these pegs? So I had a PhD student, Julio Casale who, who did this experiment and what he did was he put chicken wire all over a wall and chicken wire all over the floor as well. And he got rats to kind of wander around, um, either on the floor or on the wall. So when they're on the floor, their body was oriented horizontally. And when the rat kind of went over to the wall and climbed onto the wall now as body was oriented vertically. And what he found was that now that the rat's body is oriented vertically, as it moves over that wall, we see the grid cells are producing firing fields that are circular just like in the same way that they're circular on the floor, except that they're bigger. And there are fewer of them and they're more widely spaced. So it's almost as if there's a, there's trying to be a grid pattern on the wall, but it's very expanded. So the fields have gotten bigger and the spaces between the fields have gotten bigger, and that means you can't fit very many of these fields on walls. So there's maybe only one or two. So the same grid cell will produce a nice tight pattern, lots of fields on the floor and a much bigger pattern with bigger, fewer fields on the, on the wall.
Benjamin James Kuper-Smith:So just interrupted briefly. Isn't if I remember correctly with grid cells, you can't remember what paper that is from the Mozilla anymore, but you, uh, if you move along one direction and then trying to cortex you, they have different scalings of, uh, how big the grid patterns are. Um, is it possible that you happened when you were in the vertical dimension? Is it possible that you were just recording from grid cells that happened to have a large scaling? Or did you like really very this, I mean, yeah,
Speaker 3:No. So we would record from the exact same cell on mean Julio, Julio. This was a very difficult experiment to run. Um, so he would be recording, um, the exact same cell on the floor and then tracking it as it moved onto the wall. So the same cell could express a tight grid in one setting in the large grid in the other. Um, but you're right there, there is also this other, um, aspect of grid cells that I, that I didn't mention in your, your right to remind me to mention, which is that, um, in different parts of inter-regional cortex, the scales of this lens are different. So if you're right at the top of the brain, the scale of the grids is quite small. So the firing fields might be that say 30 centimeters parts are quite close together. Whereas if you go down much deeper than the scale gets much larger, so the fields get larger and the spacings between the fields get correspondingly larger as well. So the whole grid just gets much more expanded. So yes, there are these cells that have these different intrinsic scales, but also we see that this one cell can express different scales depending on the setting that it's in. And Julio did some analysis of some other signals and then trial called X speed signals that seem to be tracking how fast the animal's moving. And what he found was that these speed signals seem to be quite reduced. So it's as if the rat could be moving at the same speed, or let's say moving at a given speed. And if it's on the floor, um, the speed cell will respond in a particular way, but if it's on the wall, that same speed cell will respond much less it's as if that speed cell is not correctly tracking the speed. It thinks that the rat's moving much slower than it is. And so therefore everything is much bigger. It takes a take for the same amount of time. It takes it's taken, sorry, because, because the cell is treating, the rat is moving slower. It takes longer for it to get from one side of a grid field to the other. If you see what I mean. And so it's got, it's got a bigger distance. So, so that was Julio's explanation for why these, these fields are expanded. So that was different from what we saw in the paper. And we thought, well, that's sort of, um, interesting. So does that mean that the animals, not that the grid cells aren't really tracking the space, um, above the rat back. So, um, so it's tracking the surface that the animal is on and it's not interested in movement of the rat that's orthogonal, so perpendicular to that, to that surface. So then we thought, well, what would happen if the animal could move around volume, actually move freely in all three dimensions. So Rodney grieves came to my lab at that point and he, um, he took up a post-doc with me and said about trying to solve this technical problem. So this is one of these things that we mentioned that that's a natural extension and, you know, it's sort of doing something different from what anyone has done before, but it requires an enormous amount of work to make it happen. So he, um, he said about figuring out how to record wirelessly and how to track in three dimensions. So you need multiple cameras to be able to pinpoint the location of the animal and three dimensions. It's really a very tricky technical problem. Um, and he spent a couple of years solving it, and then a couple of years recording play cells and grid cells. And he found that that the place cells would produce globular place fields in this volumetric space. So as if the, the, so the, the region where the cell would become activated, if you map out that region of forms, a three-dimensional form, a globule, if, you know, what's technically called a spheroid I think, or something, but I think of it as globule, um, and these globules pur permeate that volumetric space. And we were quite pleased to see that pattern because it was similar to a pattern that, um, NACA Malinowski group had reported in bats. So in there, um, animals, these, because they're bats that can fly in three dimensions and Michael[inaudible], who was a PhD student in napkins lab had many beautifully shown that there would be these three dimensional place fields distributed throughout this, um, volumetric space. And we saw just exactly the same pattern in the rats. So even though rats and bats are, um, kind of different than how they normally explore space, their place cells seem to encode it in a very similar way, which was interesting. And then the question is what would the grid cells do? And we were still still at stage thinking that the grid cells are how the place cells are able to track the distances and therefore to know where to position their, their firing fields. So we thought that we might see, um, a lattice in three-dimensional space. So a three-dimensional grid where the symmetry of the pattern has maintained the regularity. You know, the repeating pattern is maintained in the vertical dimension, as well as in the horizontal dimension. And, um,[inaudible] group. Meanwhile, I've been doing a similar experiment and then that's so, um, PhD student, I think either a PhD student or a post-doc giddy Kennesaw, and his lab had solved this also technically amazingly tricky problem of how to record and flying bats. So really, you know, incredible work has gone into, into this seemingly simple experiment. And she had found, and they've not published this yet, but they've published several abstracts and, and shown this data at several conferences now, and we've had to talk to them about it. And, um, and they're seeing that, um, that the grid cells are forming. Uh globules so, so three-dimensional firing fields that are distributed throughout the space, but in a non regular way. So they have the same blobby appearance that we see on a horizontal surface, but, um, it's not regular. And the way that we see in a horizontal surface and then Roddy, um, in my lab, um, got the data from our rats and we saw something that's pretty similar except with was one difference, which we're trying to understand at the moment. Uh, so he again has seen the bloodiness of the firing fields of these cells, uh, and that's distributed evenly throughout the volume, but so not an, um, not, not in a, in any kind of Colombia arrangement. So clearly the cells were able to track vertical distance, but, um, it's not an irregular way. Now, the difference between, um, what the on an AUSkey group have seen. And what we've seen is that they, when they look at the statistics of the distribution of these firing fields, they see that although the distribution is the regular, the spacing is more regular than you would expect if it was random. So they see these more, um, stereotypes distances. So there's an over representation of a particular distance for a particular, it's just not precise enough to generate a regular grid, but it's there in the statistics. We have looked carefully at the statistics of our firing fields, and we don't see that in rats. And so we're trying to figure out is that because we've just not used the same statistics, although we've, we've tried to do the same type of analysis, is it that rats and bats are fundamentally different? That seems unlikely to me, what seems most likely to me is that there's a difference that's been imposed by the fact that the, the bats can smoothly and uninterruptedly traverse their environment in any direction. Whereas our rants are constrained to the directions that are orthogonal to the axis of the maze, because they're climbing through this, um, lattice like maze so that, so they have to, they can only go in certain directions. They can't go diagonally very easily, for example. So I think that that constraint is enough to disrupt the space and that's my working hypothesis, but we're trying to work our way through that at the moment with the help of some kindly anonymous reviewers. So we'll have to see what, um, what emerges from that story. Um, but yeah, so, so putting that together with the other results that we got, it looks like the exact nature of the firing fields. So the distribution of firing in different environments depends on the type of environment and the constraints that are placed on the animals movements. So on the pegboard, we saw that the grid cells didn't track height, but in the lattice we're seeing that they are tracking height. So there's something different about those two environments. And it may be to do with the ability of rats to move uninterruptedly in vertical dimension or something. I don't know exactly what it is. There's a lot of work to find out exactly what it is, but it certainly looks like the, um, the map is so as I think of it, it's out there in the world and the animals are moving through it. The map has kind of constructed on the fly as a function of what the animal can see and what it's able to do and which way it thinks it's facing and all of this type of stuff, how fast it's able to move from where that it's getting good speed information, or if that's being disrupted and so on. So it's a variety, complex, complicated web of different types of input and a variety of different maps for different settings.
Benjamin James Kuper-Smith:Yeah. I think I have a few questions here. Um, maybe I'll start with the smaller ones. One is just, uh, you said that it was quite a technical challenge to record in 3d con couldn't. You just have used the technology that a defendant in baths a few years ago and use it in rats or just, just fundamentally not.
Speaker 3:Yeah, well, that's, I mean, that's kind of what we did. So, um, so it involves getting wireless telemetry going, um, and multiple cameras. So we did the same thing, but, you know, the, the idea is not novel, but the implementation in a given lab, um, it takes a lot of work to get it, to work wireless wireless telemetry is not as easy as it sounds because the, um, the signals reflect off anything sort of metal. So you're trying to transmit with a little radio transmitter, these electrical signals, um, but they reflect off anything metal. And of course we use metal to shield our environment so that we're not picking up extraneous electrical noise, so that, so there's all sorts of technical challenges. And in your, the, if the animal gets too far away from the receiver, then the signal drops out. And, and, um, in a, in a lattice environment, if you're trying to track the LEDs on the head of the rat, um, because you've got physical struts that are creating the lattice that shoot that, that blocks off the light. So you need lots of cameras and, you know, then you need to stitch that all together and, and software and stuff like that. So it's, um, it's a non-trivial thing to get going.
Benjamin James Kuper-Smith:Okay. Um, the other question is you mentioned earlier that both Richard and John were big on thinking about what kind of things the animals would naturally do now, how three-dimensional are rats and their normal lives. I mean, it seems to me that they are quite a bit more three-dimensional than humans, for example, I mean, apart from like stairs or ladders, we pretty much are two dimensional. And, uh, I mean, I, I don't know much about, I mean, we had hamsters when I grew up, I know that hamsters, like they always like climbing and on the ceiling and everything. Right. Um, if you're in the cage, but, um, yeah, I'm just curious, like how natural of a behavior is this kind of climbing ups or through things, or, yeah.
Speaker 3:Yeah, that's a good question. And we had the same question because we thought of rats as animals that lumber around on the ground. Cause that's all we'd ever seen in the lab. So I actually went to our home office. So this country, we have like all animal researchers overseen by a government body called the home office, um, which, you know, makes sure that the ethical procedures are all tightly ahead, turns on. And they have lots of experts that they consult on various things. And so I went to them and said, you know, if I wanted to study, uh, um, um, a small mammal that would be tractable in the lab, that's got a three-dimensional ecology, uh, what would be a good species to study. And they went away and came back and said, we think the brat turns out that actually rats have a very three-dimensional ecology. So they live in these three-dimensional burrow systems, but also, um, in the wild, they do a lot of climbing. And, and in fact, I discovered this firsthand when we moved into this house that we live in currently, and we had a big Apple tree in the backyard, and I looked out the window one day just after we'd moved in and could see all this movement. And I realized that it was teaming with rats. Like there were several of them, three or four, and it turned out that I had next door. Neighbors had a colony living under their lawn. And when rat colonies get too overpopulated, the rats that are at the bottom of the hierarchy. So the ones that get bullied or violate their normal nocturnal instincts and they'll come out during the day. And so we had rats coming up into our garden and climbing up the tree and, um, you know, fighting squirrels and all sorts of entertaining things. And, and so just watching them, they, they scuttled around the branches very, very happily. So when we came to recording, um, in our three-dimensional environments, we imported into the lab, a huge, um, parrot cage. So it's a big aviary that people used to keep parrots. And that's two meters long by a meter and a half wide by, I think a meter and a half or two meters high. It's
Benjamin James Kuper-Smith:Quite large.
Speaker 3:It's very large and we've filled it with ladders and ropes and, you know, little platforms that they could climb on. And, um, and they were really happy to explore this. They're very agile and they spend a lot of time climbing. No, we'd find them all piled up in a big pile asleep on one of the platforms, for example, um, they're very comfortable climbing. Um, and so that meant that they were quite uncomfortable in our apparatus and they had had plenty of opportunity to form a 3d map and their brains if that's what they do. Um, so we're pretty sure that we are studying something that's reasonably ecologically sort of plausible. I mean, obviously there are differences. So as with a foraging on a flat surface, a rat wouldn't normally evenly four-ish through a lattice for example, but they might well explore a tree and, you know, nibble on the apples or the walnuts or something like that. Um, so I think it's not completely unnatural.
Benjamin James Kuper-Smith:Okay. That was lucky then that they said rats are not, ax has never been used for special navigation.
Speaker 3:We did also think about squirrels because there was a bit known about squirrel brain, but the problem is, you know, the thing with rats is that they've been bred for many, many generations to be tame and dose isle and not very anxious. Whereas if you use wild animals that are extremely anxious all the time, because you put them in a lab and they're as constant terror stations, it's not good for their welfare and it's not easy to work with them. And also they are very diseased. It's almost impossible to eradicate disease. Whereas, um, laboratory bread animals are completely disease free. So, you know, the, the rat to not be great for our purposes, the only problem we're having with them is that now we're starting to want to get a little bit more deep into some of these questions and look at what happens. For example, if they go upside down and rats are really not very happy upside down, um, they, because they haven't tangled with their claws and they find that really difficult. So yeah,
Benjamin James Kuper-Smith:One thing I find interesting in the video, you personally on YouTube is that whenever they want on the wall of the chicken wire, they always went down. They didn't see maybe that was a coincidence, but when I saw it, it didn't seem like they were comfortable like Chloe, like using their clothes to climb up the chicken wire. I don't know, maybe that was coincidence, but,
Speaker 3:Um, or that might've been coincidence. So, so climbing up there, not too bad climbing down, we find they tend to prefer to kind of come down backwards and sideways, so they're crab down so they can kind of see where they're going, but also they're, they're still the right way around for their claws. They don't like to be nosed down. Um, and so it's really been quite tricky. Um, and in fact, if they go nose down and they're having to just hang on with their hind feet, they, they face their hind feet back the other way so that they can use their claws like hooks as well. Mice on the other hand are really happy in any direction because they're so lightweight. So we are in the process of thinking about how to import some of their methods to mice, but then, then you have a whole bunch of other problems. Like they are so small that they don't carry a wireless device very easily. So, so there's a few technical challenges to solve there as well. So depending on the question, you know, you may need to use one species or another, or one, uh, apparatus or another, it's quite challenging.
Benjamin James Kuper-Smith:The other three-dimensional mammals, other than that's like, I'm just trying to think of any mammal that is more three-dimensional than I have or something, or male.
Speaker 3:Yeah. Squirrels probably about as three-dimensional, as it gets and chipmunks and things like that. They are zooming around blowing squirrels, playing scrolls. Yeah. Possibly. Yeah. Cause they, they fly, but they glide.
Benjamin James Kuper-Smith:Yeah. The gliders. I said that already fly that they fall slowly.
Speaker 3:That's a probably, if you want something that's truly volume metric. I think that that's probably your best bet. Um, and indeed the work that[inaudible] group has done on three-dimensional and coding in bats is just spectacular. It's it's very beautiful. Yeah.
Benjamin James Kuper-Smith:Um, I have one broader question about the amounts of dimensions that grid cells in place cells encode. Um, so I mean, you've shown that they all right. Well, I guess we also knew this from baths, but you know, your work has shown that, you know, three dimensions seems to work. Um, a one thing I'm curious about that I'm sure other people are also curious about is kind of what are the limits in terms of how many dimensions this hippocampus formation can track. Yeah. I'm just curious what your opinion is on that or
Speaker 3:Yeah. Yeah. That's a really good question that, um, people are starting to, to address experimentally now. So I mean, beyond the three spatial dimensions, you can think of other stimuli as also having dimensions, if you like. So for us, for example, color, we might also have color a color dimension from green through to red or something like that. So if you had an environment that had three spatial dimensions and one color dimension, let's say you could turn a dial and you can make your room move smoothly between green and red. And when it was green, you got one particular thing. And, um, if it was red, you got different particular thing. And if it was green, you got one type of reward in one part of the three-dimensional space. And if it was red, there was another type of wood, another type of, part of the space and so on and so on. So, um, and, and so you could take that further and say, well, not just color, but, um, all sorts of dimensions, what, you know, what they call sort of cognitive dimensions, might it be that the hippocampal system has this more general purpose function, um, and pinpointing where you are in a multi-dimensional space. And I don't know what I think about that. That's certainly the case that people have tried manipulating, um, non spatial dimensions smoothly while play cells are recorded. And they find that there are regions within that. Um, that's the minister mentioned where something that for all intents and purposes looks like a place cell will become active with it within a bounded space. So for example, our in often tank did an experiment where they manipulated an auditory tone and that a rat could kind of, as it were navigate its way through this auditory space. And there would be places within the space where a hippocampal neuron would become active. So that's quite, that's quite those types of findings are quite tantalizing. Um, so the question is, is this a really weird and a natural stimulus that the brain has decided to represent using that special module? Because it doesn't know what else to do, or is it, is this really the fact that space is just one form of a multi multi-dimensional processing capability. And three of those dimensions are used to represent three spatial dimensions, but there's all sorts of other things that could also be represented and you have time, for example. Um, and indeed, if you look at time, if you've set up your experiments, so that time has a relevant dimension, um, then play cells start to show what they call time fields in that space. So, I mean, I, I don't know what, what I think, I think it's really intriguing. I think that's the next big question to answer very intriguing, um, speculations about, um, these higher dimensional spaces and in humans, maybe that capability has been co-opted to allow us to very sophisticated, um, cognitive capabilities that we have.
Benjamin James Kuper-Smith:Yeah. I mean, that's, that's the perspective from which I'm asking. So my stuff is in humans and I mean, as, as, as interesting as the host patient navigation literature is in some sense, I don't care too much about how people get from HEB. Um, but more about like all of the things that you could represent in that, that, uh, that have a dimensionality to it. Um, and, or a continuous, um, quantity to it. And
Speaker 3:So interesting. So, so tell me more. So are you able to reveal and what your experiment
Benjamin James Kuper-Smith:Doing it directly? No, it's just, I mean, this is something, um, I mean there are Inhumans that are quite a few studies where they, I mean, Tim Barron's has been doing most of that. So a lot of the most famous stuff there. Um, and I think my point is more that I'm not working on that director right now. It's more, this is just something I'm curious about to what extent is, I mean, number one, can any dimension be encoded in the hippocampal formation and number two, how many can be tracked at the same time? So is this because so far we've only, I dunno what the maximum amount of dimension is. Anyone is tracked in a, is it three? I don't know, like at the same time?
Speaker 3:Well, I don't know. I mean, so, so yeah, I don't know, actually I was going to say that, that there are place shelves that have a, um, a place field, but also if you, when it's in the place field a few minute plates, some of the stimulus you can, um, get firing, um, within the bounds of that stimulus as well. So the cell will only fire if the animal is in that place and experiencing this other stimulus, for example, but having said that that's been done in two dimensional spatial environments. So I guess the maximum dimensions is still three. So the question is could in a three-dimensional environment, could you have a four, four dimensional? Yeah. And I think it's quite possible quite possible because they have a campus does do a lot of associating and play cells do seem to be very interested in tracking movement through some continuum relative to some fixed point. So, um, yeah, I guess we will find out.
Benjamin James Kuper-Smith:Yeah. Um, I w I have two more points, but I don't think we'll get through it without making this too long. Um, I mean, like, one thing I was interested in is, uh, um, so as I wrote in your, uh, you know, as a rich in my email to you, like I had read[inaudible] Valley's books or one, this, the seven brief lessons in physics when I was called, I thought it was really cool. And, uh, you know, it's just like a thing I read once. And then for some reason I saw, you know, Kent Jeffrey has a paper with, um, I can't remember, I think it was just the one with all Valley and I thought, okay, it's something on entry, whatever. And it took me a while to realize that that's the same person, never hit those physics. Like I didn't, you know, it's you, that that was the same person. So, um, I mean, I, your trends and your essence paper, I really loved these kind of papers that kind of make you think of like the big scale of things. Um, and in a way I'd like to talk about that in depth, but I want to read like lots of papers before I do that. So I'm not capable, but now
Speaker 3:I'm writing a book on that, on that subject. So maybe you can read that.
Benjamin James Kuper-Smith:Awesome. Very happily read it. Is that tentative publication date for that? Or is it
Speaker 3:Well, no, it's it's, we were way off in a year probably.
Benjamin James Kuper-Smith:Um, but then, uh, I'm just curious, like, how did this cross-disciplinary collaboration get going?
Speaker 3:Yeah. So this, this came about, so sort of by, by chance. So I was invited to a, um, a very elite discussion meeting. I was very privileged to be invited to this discussion meeting that was organized by URI Zaki, who is another luminary in my field, who is very interested in, um, things like brain oscillations and, and aspects of processing, I guess, that devolve space and time. So he, he organized us a discussion meeting that brought together neuroscientists, who work on space and time together with physicists who work on space and time. And we were all closeted in a, um, resort in Costa Rica of the most amazing experience to be in this stunningly, beautiful location with this small and extremely knowledgeable set of people. And I'd been really excited to meet the physicists. I mean, I knew most of the neuroscientists, I was happy to meet up with them again, and you all of the neuroscientists, but I hadn't met the physicist. And there were, you know, people like Sean, Carolyn Carter color Valley, who I knew because of the popular science, but were outside my research area. Um, and I had sort of, I'd been thinking, what am I going to talk about? Um, how could I possibly have anything that's of any interest to any of these amazing people? Um, and, and my talk was on the third day. So there were three days of talks and the first day was from the physicist and they were talking about how they think about space and time. And it was all very relativistic and quantum and Steve with, you know, big picture questions like, like entropy and complexity and things that I just find super cool, but, you know, quite a long way removed from what I do in my daily life. And the second day was the neuroscientists. Um, w w was neuroscientists, not all of us, because some of us were on the third day and the neuroscience, you know, explained to the physicists all about grid cells and place cells and memory, and, you know, how we all think of this, um, how, how it works. And it was all that was all called swell and the physicists in really interested. But, um, and also since that, there wasn't really a lot of overlap between what the physicists had talked about on day one and neuroscientists talked about on day two, and also I felt like we'd had an awful lot of grid cells and play cells and very esoteric, um, neuroscience on day two. And I just thought, I just don't feel this meeting needs more of that because I didn't really have anything much to add other than the stuff I've been doing on three-dimensional processing, which is, you know, not, not uninteresting, but I just thought, I sort of wonder if I can make better use of this brief opportunity to talk to these amazing people. Um, and I, so, so I turned to thinking about something else that I had been really pondering on a lot as an ruminating on for the last year or two, which is sort of to do with the climate crisis, which, you know, in 2018 and, and more so in 2019 started to really come to public attention because we had, um, the series of freak weather events, super hot summers wildfires, you know, super freezers, you know, amazing floods, like super extreme heat waves that were killing lots of people in and tropical regions. And so a series of calamities and around about this time, um, environmental activists started to get really vocal and say, look, we've gotta be doing something. And I was paying a lot of attention. And I felt, in fact, I joined extinction rebellion, um, because I felt like they were the biggest opportunity to get the attention of politicians. Nobody else seemed to be succeeding and getting the attention, but these people seem to be succeeding. And they had a lot of very creative and innovative ideas. So I joined them and started reading up about the climate crisis and, um, really getting quite depressed about the future of humanity, really, which seemed to be quite grim and rethinking, or my, you know, happy optimism about how science is going to take us forward into the greater means in the future and thinking, actually it looks like we might be driving ourselves into extinction and reading it, reading up about, um, climate over paleontological time, trying to understand how we got into the situation and, and just thinking about, you know, really big questions to the evolution of the universe and the evolution of life. And, um, how does this fit in with entropy? Like how did, how did life come to be, how did we become so complex and so amazing on the one hand and yet be about to drive ourselves into extinction? How, um, how does this all work scientifically and, and how does that fit with entropy? And my understanding of entropy was that really, it involves the, um, the constant creation of disorder. You know, things break eggs, break things, don't spontaneously form, you know, a house of cards, collapses, the houses of cards done spontaneously form, and you're on the one hand, on the other hand, life spontaneously formed is life, some type of reversal of entropy. And I was familiar with, um, Schrodinger's work, you know, shredding the physicist who gave us the uncertainty principle. And so, and I was familiar with his, his musings on the subject, back in the 1940s. And he had speculated that life was somehow able to reverse entropy on a local scale. So clearly you can't reverse entropy on a global scale that laws of thermodynamics are the laws. You know, they can't, they can't, they're immutable. The universe is running out of free energy, but life can sort of temporarily roll back on that by borrowing entropy from its surroundings, if you like that uses energy to kind of reverse entropy. That was my understanding of what Schrodinger was saying. So, so I presented that. I decided I was going to present this to this physicist. And I spent the whole night before my talk, I'm writing this completely new talk from the one I had intended to write, and my heart was in my mouth. And I thought, I've got, this could be career ending. You know, um, I've gone way above my pay grade. I'm talking about all of these things. I know nothing about, it's not what I was brought here to talk about. This is possibly a terrible mistake, but, you know, humanity is headed for extinction. So I don't care. This is my one chance to talk about the climate crisis, all of these people. Um, and so I did, I, you know, my opening slide was, you know, um, the fate of complexity in the universe and I put this up there and the whole room erupted into laughter and then applause. And I thought, Oh man, maybe this is going to work. I don't know. Um, but at that stage I was full of adrenaline and I thought, I don't care. I'm going to, I'm just going to do it. And so I laid out this whole problem from start to finish. This is how the universe unfolded and, you know, harking back to the talks we'd had on day one. And, um, Sean Carroll talking about complexity and how it at first increases and then decreases and Carla Ravalli talking about entropy and so on and time, and this was all weapon. And I said, okay, so the physicist told her this, this, um, but my, my understanding as a biologist, um, is this and I, and I went through the evolution of life and the evolutionary transitions. And these are the things that I then talked about in this paper that you've read, um, how, how there seem to be these step changes is step increases in what life can do. How does that
Benjamin James Kuper-Smith:Question here? Did you know, all of that already? Because to me, this was like one thing that I found so cool reading. This was like, these are all these things. I've I, I mean, you know, I just didn't, I've never read any of those papers or anything like that, right? Yeah, no. So, so at that
Speaker 3:Stage, I was still of the view that life is a reversal of entropy, um, that I had the shredding of you life manages to temporarily reverse entropy by borrowing it from its surroundings. But there was a, there was an explanatory gap there, like, how does it do that? What makes it do that? Why does complexity seem to go against entropy? And yet complexity seems to inexorably increase over evolutionary time. Like we evolved from single cell organisms to societies of humans, which, you know, we, we like to think we're very complex. I think, I think it's not unreasonable. Um, but, but, you know, why does complexity sensitive all from one direction? You know, like there's, there's a, there's a, there's a gap there. So I laid that out to the audience. I said, what's the gap physicists helped me out here. Um, and so Carlo Ravalli and Sean Carroll, you know, collared me afterwards and said, you know, we, we think we can help. And, um, so, so I had some really good conversations with them, both separately and also together. And indeed Sean, um, asked me, um, onto his podcasts subsequently so that we could have that same conversation in public as it were. Um, and I would articulate the same problem I was having as well as just then he would give to the physicist view. And it turns out physicists don't really have necessarily all the answers either because when they think about entropy, um, they tend to think about an ideal gas in a box. And I'm really, some of the notions do get a bit fuzzy when you're talking about highly complex systems, but the second law of thermodynamics nevertheless runs through everything. And that is a, um, but the really big insight came from Ravalli. And he said, look, you've, you've got it wrong about life reversing. Entropy life is not reversing entropy life as an entropic process. The mistake that you've made that trolling a made and lots of other biologists have made is equating order with entropy. So while it's true, that entropy often creates disorder, entropy can also create order. And then he gave some sort of examples of non-living things where order arises spontaneously. And one of them is if you start off with shaken up oil and vinegar and a jar, if you let that unfold over time, they will separate out into a more orderly arrangement where the vinegar, where the oil is on the top and the vinegar is on the bottom. So actually you can get order from entropy, um, and that is able to happen because of things like gravity. So when you've got gravity in the mix, if we didn't have gravity, um, you wouldn't get that separation, but because we've got gravity, you do. Um, and slowly, you know, it took me a while to work through all of this in my head and, and start to understand it. But slowly, it began to make sense that you can get complexity because complexity is the formation of order and it's coming about because of, um, entropically driven processes, working together with some other processes like gravity and so on. So we wrote that. So, so Carla emailed me and, um, another colleague Robert and said, shall we put this all together into a paper? So he wrote that paper that was published in this journal entropy. Um, and, and so he wrote, he wrote the, the bones of it, and then Robert and I kind of contributed to our biological view and made it comprehensible to biologists, don't dance on mathematics and, um, and, and kind of related it to the process of evolution and genetics and so on. But then I felt that there's more to be said on the subject to do with evolutionary transitions in the, in the formation of the nervous system. So, so then I wrote the second paper as well. And so, and sort of outlining that same concept about how entropy can drive the development of complexity, and that can explain why evolution generates more and more complex organisms over time, but also how we can sometimes face extinction. So one of the big facets of evolution, there's constant extinctions. So complexity doesn't always increase. It's a statistical process. And just because we're here doesn't mean we will always be here. And the D if you look at the history of things that have evolved in the past, they usually do extinguish themselves. So then that's a challenge for us as humans. Um, can we, can we be clever than that and stop our own extinction using a highly complex, highly evolved brains? And that's the, that's the big challenge for us.
Benjamin James Kuper-Smith:Okay. Yeah. Yeah. That said, like, I, I just, I really enjoyed reading the paper, but I don't have, um, uh, I think I need to read a lot more and should, I can make some comments, it physically specific questions, but I guess I'll wait then impart until your book comes out and just read that then. Yes.
Speaker 3:I mean, if there are things that you were puzzling over, then please let me know because, um, if you were passing over them, if you haven't listened to the podcast with Sean Carroll, um, you could do that. Or you could look at the transcript, which might be a bit quicker because you may see that a lot of the questions that you have are the ones that I had to, that I asked him. So the way that we did that podcast is a, it's more of a Q and a, um, type of, um, backwards and forwards. So I interviewed him as much as he interviewed me. It was just a slightly unusual format for a podcast, but, um, we thought that would be the way to do that. And then I think it did cover a lot of things that people like me have wondered about. And then if you've got questions beyond that, or indeed, um, aspects of that, that you thought were particularly salient or, uh, hard moments for you, uh, let me know. Um, then I'd note to focus on those.
Benjamin James Kuper-Smith:Yeah. Okay, cool. And last, not even question, but maybe more comment. Um, so one thing I also found is that to me, this just the title, right? Transitions in brain evolution, space, time, and entropy somehow. I mean, space and time and praying. I can, I can very obviously see how that relates to your work, but the other thing didn't quite, it seemed like kind of left field to me. But then the funny thing is one of the first things you mentioned when we talked is that you really liked, um, information theory, um, early on, is that kind of a, um, almost you pursuing interests almost you had from the very beginning of your
Speaker 3:That's, right? Yeah. Closing the loop, um, that only really occurred to me quite recently, actually, that that's exactly what I've done. I've found my way back to inflammation theory. And I guess, you know, I guess my sort of interest in neural coding and how the mind can arise from the brain is sort of related to these bigger questions of how does complexity arrive arise for nothing, you know? And that they're just super big questions and I've always been drawn to the really big questions. Um, and now this is trying to just tie it all together, I guess. Um, the one thing we haven't solved is consciousness. I think that's possibly for a different lifetime.
Benjamin James Kuper-Smith:Yeah. Yeah. Whoever solves that one is up for now,
Speaker 3:I'm not sure that there is a solution. I sometimes think that the, the question of consciousnesses is like the old question of life. You know, we used to wonder where life came from in living things. And there were these ideas that there was this vital force that flows through living things. We don't ask that question anymore because we now can see that life is just a emergent property of very complex systems that have, you know, movement and so on. I suspect that we will just come to the same conclusion with consciousness that is sort of not a meaningful question. It's just a natural emergent property of neurons doing what they do. And there, isn't a thing to be, to be explained if you see what I mean.
Benjamin James Kuper-Smith:Yeah. I know. Yeah. I mean, and I've heard the argument that it's not exactly as soon as we had the double helix for DNA, that basically once that came out the question of like, what is life suddenly, um, yeah. Became less relevant. That isn't, that isn't that basically what Daniel, Dan is saying almost, I can't remember. I've I've done fairly little about consciousness, but I thought he said basically like what his position is that kind of what you just said? Like once you actually find the mechanisms, the whole program kind of just disappears almost.
Speaker 3:Yeah. Yeah. There isn't a ghost in the machine. Yeah.
Benjamin James Kuper-Smith:Yeah. Well, we'll see. Good luck to whoever wants to sell that one, make it go away.